This post is a summary of the findings of a paper co-authored by researchers from the DLT Science Foundation and others, including the author of this post.
Blockchain technology has been at the epicentre of the technological revolution of the 21st century. Enabling large efficiency gains, new decentralised applications, and even the denationalisation of money, blockchain has positioned itself as one of the key innovations shaping the IT landscape.
However, blockchain has also been in the public eye due to an increasing outcry concerning its environmental impact.
Specifically, Proof-of-Work (PoW) consensus algorithms (when in combination with small blocks and long block times, as is the case of Bitcoin Core) have been denounced for the high energy consumption to which they lead, especially in the case of Bitcoin.
A significant debate has raged therefrom, on many dimensions: the correct metric to measure energy consumption (per transaction, per cumulative transactions, per USD of value secured, per BTC of value secured, etc.), the extent to which bitcoin are mined with renewable energies, the potential of Bitcoin as a flexible load resource that may stimulate renewable penetration and even net decarbonisation, and more.
Proof-of-Stake vs Proof-of-Work
In spite of this, a consensus has remained on a particular fact: Proof of Stake (PoS), an emerging alternative to PoW, consumes significantly less energy than PoW.
For many of those concerned with energy consumption as a proxy for carbon footprint (the limitations and nuances outlined above notwithstanding), this has acted as a selling point.
The bad press of PoW has nevertheless been argued to hurt PoS blockchains, as the technological differences between different blockchains are not always perceived by the public, leading to all blockchains being “put in the same bag”.
Many efforts have been undertaken to try to differentiate PoS from PoW. However, the energy consumption of PoS remains an under-researched topic.
As a result, we have limited precision on the exact extent to which PoS is less energy-intensive than PoW, as well as on the differences between the many PoS-based blockchains on the energy front.
Early Attempts at Measuring PoS Energy Consumption
In 2021, Platt et al sought to begin to fill this gap, by first collecting data and approximating a methodology that could enable comparisons between many PoS systems.
This was not restricted to just blockchains, but DLTs (Distributed Ledger Technologies) in general - the wider category of decentralised networks that encompasses those organised in chains of blocks.
The main findings can be summarised in the following figure:
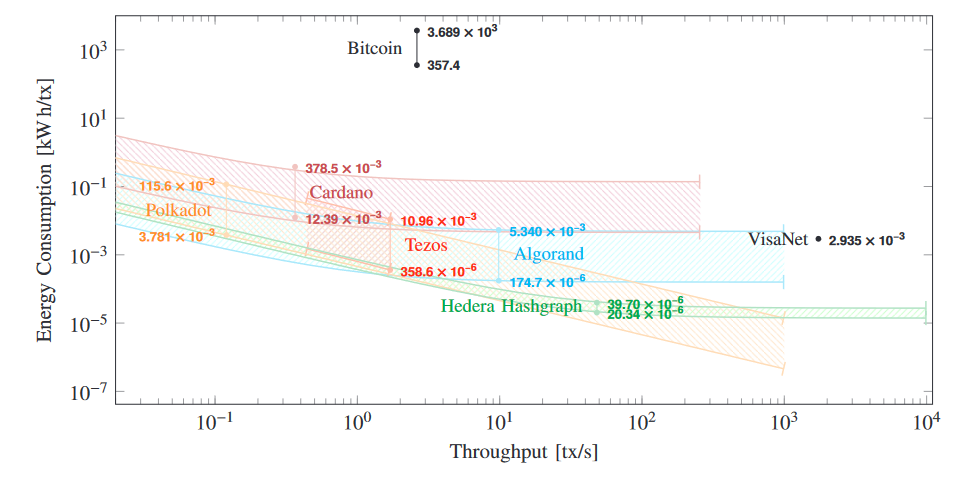
Although simplistic, the methodology of this work can be regarded as pioneering because of a transparent method with a few interesting features.
Notably, it controls energy consumption per transaction for throughput, meaning the authors are aware that simply comparing energy consumption per transaction is not enough of a denominator, as the value of this variable can be very different at different levels of throughput, and indeed different levels of throughput underlie any empirical observations.
Some have addressed this by making measurements in a lab setting. Still, the authors choose instead to prioritise real-world observations and extrapolate from them a function describing how energy consumption per transaction evolves with throughput, a novel approach.
The function for each DLT is illustrated in Figure 1, with the lower and upper bounds representing optimistic and pessimistic energy consumption assumptions for the hardware used.
This work is pioneering, but of course still embryonic.
A New Proposed Methodology for Measuring PoS Energy
With this in mind, in a recent paper, researcher Francisco Rua and I sought to develop this further.
In our working paper “The energy consumption of Proof-of-Stake systems: Replication and expansion” we replicated the methodology of Platt et al (2021), and further expanded and updated their work.
We included additional DLTs among our study objects. To the original paper’s Algorand, Cardano, Ethereum, Hedera, Polkadot and Tezos, we added Avalanche, Elrond/MultirversX, Flow, NEAR, Tron, Toncoin, and Solana, hence covering the entirety of the layer 1 (L1s) PoS DLTs in Coinmarketcap’s top 50 ranking by market cap.
We also took real-world observations for Ethereum (Platt et al had relied on pre-Merge estimates), replicated all observations, and included additional, more up-to-date observations.
Finally, we incorporated more granular research on the hardware used by each DLT.
Our findings were as follows:
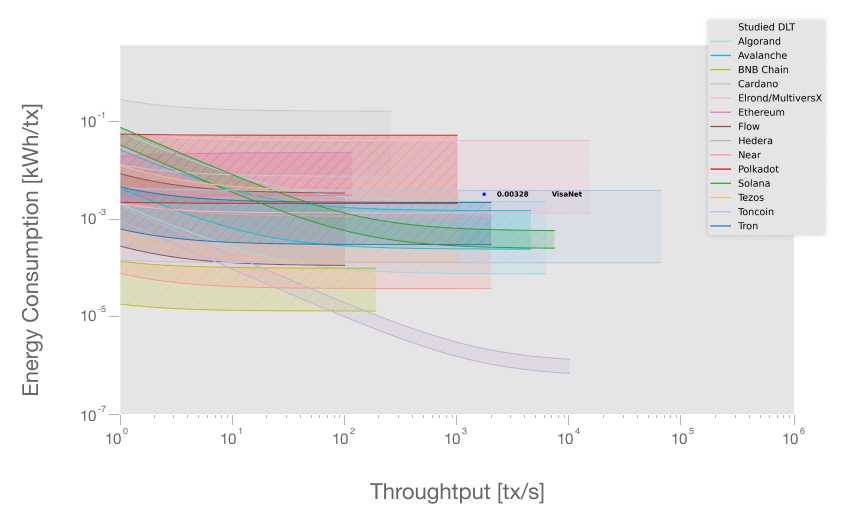
Figure 2 provides the best comparison of the DLTs under review, and we encourage usage of this figure.
In addition to the graph, we also share the details of our latest observations:

What we can learn from this is the following:
- PoS DLTs energy consumption can be studied and is indeed significantly lower than Bitcoin Core’s energy consumption.
- Furthermore, PoS DLTs have energy consumption levels that are on par with VisaNet’s, if not lower.
- PoS DLTs indeed do not display all the same energy consumption levels, with differences not just between networks, but also between each network depending on the level of throughput at issue.
Read the Full Paper
We encourage you to read the full working paper, where you will find a detailed explanation of the methodology, limitations, and our interpretation of the findings. We will continue to update this article as we collect more observations.
In line with the open decentralised spirit of the DLT ecosystem, here’s a link to a Github repository that can be used to replicate our entire methodology, as well as improve it and continue to update it and expand it yourself.
Did you spot a way to improve our work? We want to hear from you; please get in touch and let’s talk.
References
1 https://scholar.google.com/citations?user=vspNXLUAAAAJ